Research
Next-Generation Rechargeable Battery Technology (Energy Storage)
Development of the Novel Electrolyte with Unparalleled Design Strategies
Electrolyte solutions in lithium-ion batteries are lithium-ion conducting solutions enabling lithium-ion transfers between positive electrodes and negative electrodes. Because they are generally produced by mixing lithium-salts and organic solvents, conventional electrolytes have been designed based on the two parameters, the kinds of electrolyte salts and solvents.
Our group has developed a novel electrolyte design strategy focusing on controlling ion-solvent coordination environment in electrolytes, which is different from the conventional design strategy. For example, we have demonstrated controlling the various kinds of electrolyte properties such as expansion of voltage stability, unique passivation reactions, peculiar ion-transport properties, and prevention of current collector corrosion in positive electrodes by adjusting the concentration of traditional electrolyte salts and tuning the coordination environment of lithium-ion and solvents. Furthermore, we have focused on the molecular structure of electrolyte solvents. The non-flammable phosphate ester solvent, which is incompatible with graphite negative electrodes, was converted to the cyclic phosphate which has the similar structure to that of the conventional ethylene carbonate. This molecular design enabled the reversible charge-discharge of graphite negative electrodes. These results suggested that the cyclic phosphate is an alternative safe solvent to the ethylene carbonate. Our group aims to develop novel electrolyte materials based on the unparalleled design strategies focusing on the three viewpoints of salts, solvents and ion-solvent coordination for leading the innovation of energy storage/conversion devices.
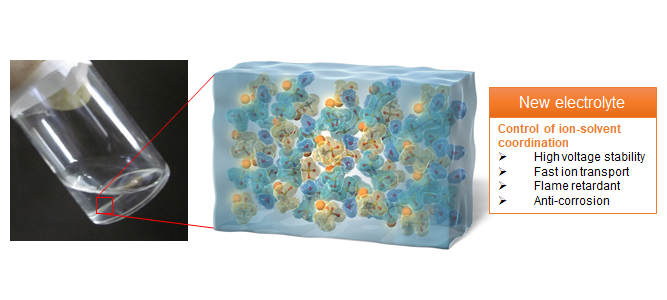
<Related works>
Nature Energy 5, 291-298 (2020).
Nature Energy 3, 22-29 (2018).
ChemElectroChem 2, 1687-1694 (2015).
Journal of the American Chemical Society 136, 5039-5046 (2014).
Pioneering the Novel Reactions for Next-Generation Energy Storage
Commercialized lithium-ion batteries are constructed utilizing layered transition metal oxides as positive electrodes, graphite as negative electrodes and organic electrolyte solutions as lithium-ion conductive electrolytes. Current lithium-ion batteries already possess close values of energy density to the theoretical values. Increasing battery voltages and electrode capacities by development of novel electrode materials or new battery reactions are demanded for obtaining higher energy density.
Our group seeks to create novel reactions enabling high density energy storage due to high-voltages and high-capacities. It is much significant to study both electrode and electrolyte materials for constructing high-voltage secondary batteries. For example, when high-voltage charging is conducted for lithium-ion batteries containing conventional electrolytes and high-voltage positive electrodes, the batteries face severe degradation during battery cycling due to the dissolution of positive electrodes and/or the electrolyte decomposition on electrodes. Hence, it is desired to prepare electrolyte solutions stabilizing the positive electrodes and being stable under the high-voltage. We invented new electrolyte solutions produced from the conventional linear carbonates and the super high concentration lithium salts with high solubility and high chemical stability. These super-concentrated electrolytes solved the problems mentioned above and enabled the charge-discharge cycles of 5 V class high-voltage lithium-ion batteries. Furthermore, for high-capacity lithium metal negative electrodes, we achieved its unprecedented high charge-discharge efficiency by utilizing rational electrolyte design. Our group aims to pioneer novel reactions for high density energy storage through studying both electrode and electrolyte materials.
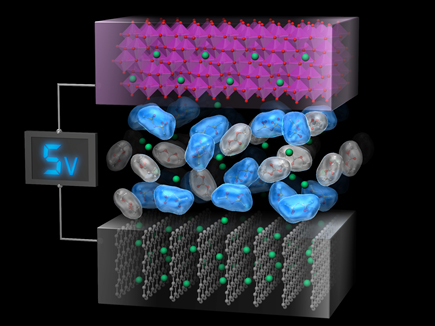
<Related works>
Joule 5, 998-1009 (2021).
Angewandte Chemie International Edition 58, 8024-8028 (2019).
Energy & Environmental Science 10, 1828–1842 (2017).
Nature Communications 7, 12032 (2016).
Development of the Next-Generation Rechargeable Battery Technology
Lithium-ion batteries have been widely used for portable electronic devices because of their high energy density. Recently, lithium-ion batteries have been also applied for large energy-storage devices such as electric vehicles, and there is a large demand for increasing their energy density. The pioneering novel electrode materials or new battery reactions are desired for producing batteries with high energy density. Furthermore, in terms of battery safety, development of non-flammable electrolytes is necessary to minimize the risk of battery fires and explosions especially for the large-scale use.
Our group aims to develop next-generation energy-storage devices solving those battery issues. For example, we invented novel lithium-ion conductive aqueous electrolyte, “hydrate melts” utilizing the eutectic system of two kinds of lithium salts. It is well known that usual aqueous lithium-ion batteries containing non-flammable aqueous electrolytes cannot be charged to the high voltage regions of conventional lithium-ion batteries owing to the low voltage stability of water. On the other hand, we succeeded to construct non-flammable 3 V class high-voltage aqueous lithium-ion batteries by utilizing the “hydrate melts” as electrolytes. Our future work will focus on pursuing the limit of such new aqueous batteries and inventing innovative secondary batteries due to seeking novel materials and reactions.
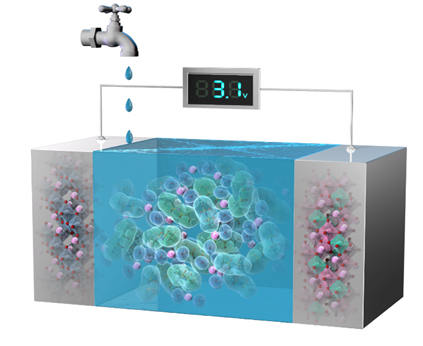
<Related works>
Electrochemistry Communication 116, 106764 (2020).
RSC Advances 10, 4129-4136 (2020).
Electrochemistry Communication 100, 26-29 (2019).
Angewandte Chemie International Edition 58, 14202-14207 (2019).
Nature Energy 1, 16129 (2016).
Analysis of Charge-Discharge Reaction Mechanism in Secondary Batteries for Fast Charge-discharge Reactions
Recently, electric vehicles (EVs) utilizing lithium-ion batteries have been commercialized. To develop higher-performance EVs, rapid charge-discharge is strongly required for the batteries. Charge-discharge reactions of lithium-ion batteries can be simply explained as the reversible shuttle of lithium-ion between positive and negative electrodes. However, internal resistances in lithium-ion batteries are actually derived from several reaction steps. Hence, there is a large demand to understand the charge-discharge reaction mechanism in lithium-ion batteries for reducing the internal resistances.
Our group aims to fundamentally analyze the origin of the internal resistances and the reaction mechanism in lithium-ion batteries. It is well known that the energy barrier in an interfacial lithium-ion transfer at solid/liquid interface between electrodes and electrolyte solutions is significantly large in most of the lithium-ion batteries, which is the large obstacle for the rapid charge-discharge. We clarified that the energy barriers of interfacial lithium-ion or sodium-ion at electrode/electrolyte interfaces depend on the electrolyte compositions. We will elucidate the reaction mechanisms of various rechargeable batteries, propose a clear guideline for accelerating the reactions, and develop next-generation energy-storage devices with rapid charge-discharge rate.
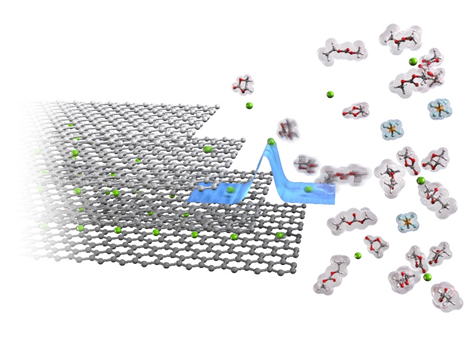
<Related works>
ACS Omega 6, 18737-18744 (2021).
ChemSusChem 13, 4041-4050 (2020).
Journal of Physical Chemistry C 113, 14528-14532 (2009).
Breakthrough Fuel Cell Technology (Energy Conversion)
Breakthrough Energy Conversion Device with “Single-Atom Catalyst”
Noble metal-based catalysts loaded on conductive support are gold standards for catalytic processes at the heart of electrochemical material conversion devices. Supported metal catalysts are often most effectively utilized when the metal is dispersed as extremely small particles - and in the limit as isolated metal atoms. Reaction pathways on isolated metal atoms can be very different from those favored on metal nanoparticles.
Our group explore synthetic approaches for creating supported single atom catalysts, site specific techniques to characterize their structure, and operando analysis to understand reaction pathway. One of the goals of this research is to understand how metal atoms and few-atom metal clusters catalyze certain electrochemical reactions. Furthermore, we aim to establish the strategies to tune their reactivity through metal-support interactions to enable unique catalytic activity for high-efficient energy conversion devices. All of these experiments fit together to make breakthrough in electrocatalysis to address critical energy problems across the globe.
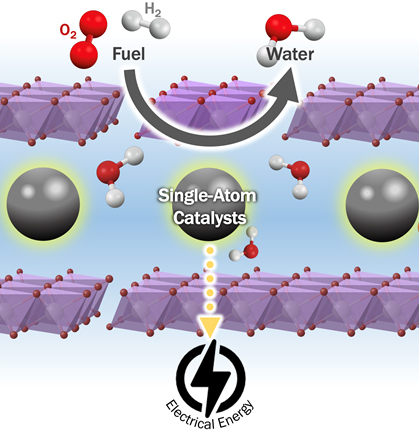
<Related works>
ACS Applied Materials & Interfaces 13, 28098–28107 (2021).
特願2021-040510, PCT出願
Innovative Power-to-X Technology (Electrosynthesis)
Revolutionize the Electrochemical “Power-to-X” Technology
With the emergence of abundant renewable electricity, industries are replacing conventional thermochemical routes with electrification to reduce carbon intensity. However, electrochemical material conversion faces challenges, as conventional thermochemical routes are often not easily electrified. Furthermore, highly active and state-of-the-art, high cost and scarcity of noble metal-based catalysts necessitates the development of alternative (electro)catalysts. Therefore, we are developing precious-metal free catalysts for efficient electrocatalytic reactions to synthesize chemical fuels and feedstocks using renewable electricity, abundant resources, and water.
Our group seek to apply this approach to a variety of different chemistries where electrochemical routes are promising, such as the conversion of carbon dioxide into chemical feedstocks (e.g., methene), water electrolysis to convert water into hydrogen and oxygen, and electrochemical ammonia synthesis that can replace energy consuming conventional Haber-Bosch Process. Our studies on electrocatalysts encompass extensive experimental components, e.g., the operando investigation of surface and interfacial processes using advanced characterization techniques. Our group aims to integrate fundamental understanding of catalyst materials into industrially-relevant electrolyzer systems.
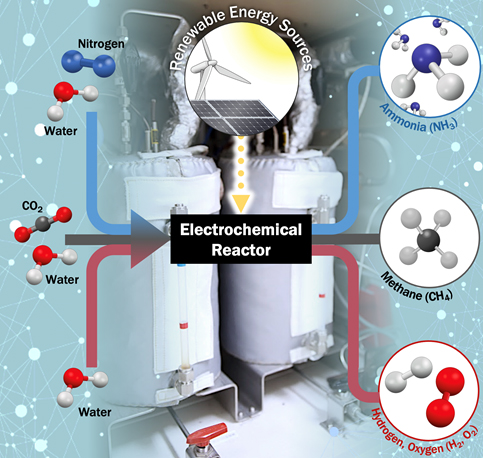
<Related works>
Nature Catalysis 3, 516–525 (2020).
Journal of Physical Chemistry C 123, 5951−5963 (2019).
Science 358, 751–756 (2017).
Exploring the Novel Reactions in the “Electro-Nanoreactor”
Beyond bulk metal catalysts, we have employed host-guest layered materials as platforms to design electrocatalysts that combine the tunability of molecular catalysts with the practical advantages of heterogeneous catalysts. Host-guest layered materials are a class of hybrid materials constructed from metal oxide layer (host layer) and compound with cationic nature (guest layer). Compared with traditional heterogenous electrocatalysts such as metal alloys, host-guest layered materials possess unprecedented tunability by modifying components within guest layer. We have demonstrated that the guest layer can contain metal complexes, sub-nano metal particles, and hydrated cations, which can be utilized as the electrocatalyst by the electron transfer through host metal oxides. Therefore, the guest layer can be considered as tunable electrochemical nanoreactor, which contains both reactant and electrocatalysts. Future works will focus on the design of the electro-nanoreactor, which then implemented in a variety of electrocatalysis including oxygen reduction reaction, water splitting, and CO2 reduction reaction.
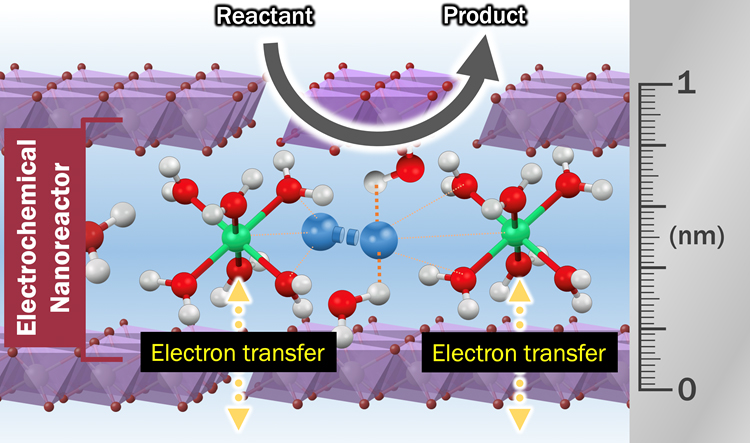
<Related works>
ACS Applied Energy Materials, Submitted.
Pioneering “Advanced” Electrochemistry
Establish “Liquid-” Materials Chemistry
Electrolyte solutions produced by the dissolution of electrolyte salts such as lithium salts to solvent medium such as organic solvent has been applied in various devices utilizing electrochemical reactions. Theoretical analysis in chemistry and electrochemistry about liquid materials such as electrolyte solutions has been aimed for mainly dilute electrolyte solutions. On the other hand, the theoretical understanding for electrolyte solutions with high salt concentration has not been achieved because such concentrated electrolyte solutions have not been paid attentions due to the disadvantage of low electric conductivity etc.
Our group has demonstrated controlling the lithium-ion coordination environments in electrolyte solutions and electrolyte properties due to increasing the salt concentration. The chemical understanding of liquid materials including the salt concentrated electrolyte solutions must be promoted. In addition, electrolyte solutions at the electrode/electrolyte interface, where electrochemical reactions occur, possess different structures and properties compared to the usual bulk electrolyte solutions. Hence, the scientific insight about the electrolytes at the solid/liquid interface must be also deepened. In this context, our group aims to establish novel “-liquid-”materials chemistry/electrochemistry about various electrolyte solutions. Furthermore, our future work will focus on the design of novel electrolyte materials and the development of innovative energy storage/conversion devices based on the future developed theory.
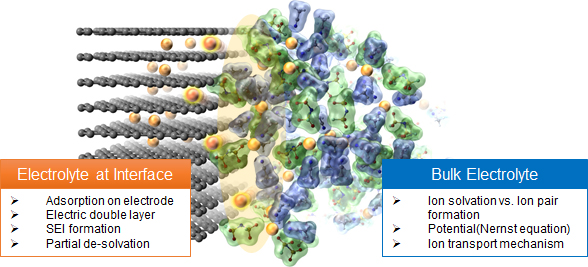
<Related works>
Journal of .the American Chemical. Society. Au 1, 1674−1687 (2021).
Nature Energy 4, 269-280 (2019).
Bulletin of the Chemical Society of Japan 93, 109-118 (2020).
ACS Applied Materials & Interfaces 11, 35770-35776 (2019).
Journal of the Electrochemical Society 162, A2406-A2423 (2015).
Design and Analysis of the Electrode-Electrolyte Interface
(Electro)catalysis provides exciting opportunities to address some of the impending global changes of the 21st century ranging from energy and environment to food and chemical production. The unique catalytic activity of electrode stems from its distinctive geometric and electronic structure, that can be tuned to facilitate numerous (electro)catalytic process on the surface, including insertion, adsorption, conversion, and desorption of reactant and product molecules in organic/aqueous media. Although the electrocatalytic activity has been improved by tuning the electronic structure of the electrode materials, there has been little focus on engineering the electrode/electrolyte interface. Furthermore, little is known about molecular processes and interactions that govern reaction efficiency, activity, stability, and selectivity, which limits the design of new functional electrode/electrolyte interfaces. Therefore, there are immense opportunities for fundamental research of electrode/electrolyte interfaces to identify governing parameters that dictate the complex interactions at interfaces. Our group aims to connect the understanding of surface reaction mechanisms obtained by in situ/ex situ spectroscopic techniques combined with theoretical analysis to activity and stability of the catalysts, as well as to establish novel design principles for designing electrode/electrolyte interfaces.
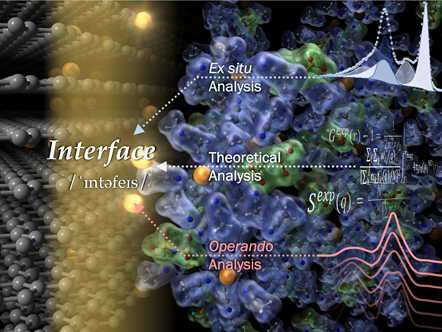
<Related works>
Energy & Environmental Science 13, 183-199 (2020).
ACS Applied Materials & Interfaces 11, 45554-45560 (2019).
ACS Catalysis 6, 2026–2034 (2016).
Chemistry Letters 46, 1056-1064 (2017).
Journal of the American Chemical Society 136, 5039-5046 (2014).