[ Ratiometric Bioluminescent Indicator for Simple and Rapid Diagnosis of Bilirubin ]
( Yukino Itoh, Mitsuru Hattori, ACS Sensors )
Unconjugated bilirubin (UCBR), a metabolite of hemoglobin, tends to reach a high concentration especially in newborns, which causes newborn jaundice and nuclear jaundice (bilirubin encephalopathy). Recently, jaundice has become easy to be severe with the increase in the number of preterm infants. Therefore, a highly sensitive UCBR measurement is needed for the early diagnosis. We have developed a bioluminescent UCBR indicator, "BABI” (Bilirubin Assessment with Bioluminescence Indicator), that enables a simple and rapid UCBR concentration measurement in small amounts of blood. Since the bioluminescent color of BABI changes so clearly that it can be visually confirmed depending on the UCBR concentration, it can be captured by using a versatile device, such as a smartphone camera. We demonstrated the UCBR concentration measurement in a small amount of whole blood using mouse blood spiked with UCBR to emulate human newborn blood. This method requires only mixing 3 µL of blood sample into BABI and taking the bioluminescence image by a smartphone camera as a measurement procedure. The high affinity to UCBR and the large dynamic range of BABI make the highly sensitive diagnosis possible even before the symptoms of jaundice appeared on the skin and eyes. This assay with BABI is expected to be developed as a measurement platform for point-of-care diagnosis in the future, as it can be performed anywhere and requires no specialized skills.
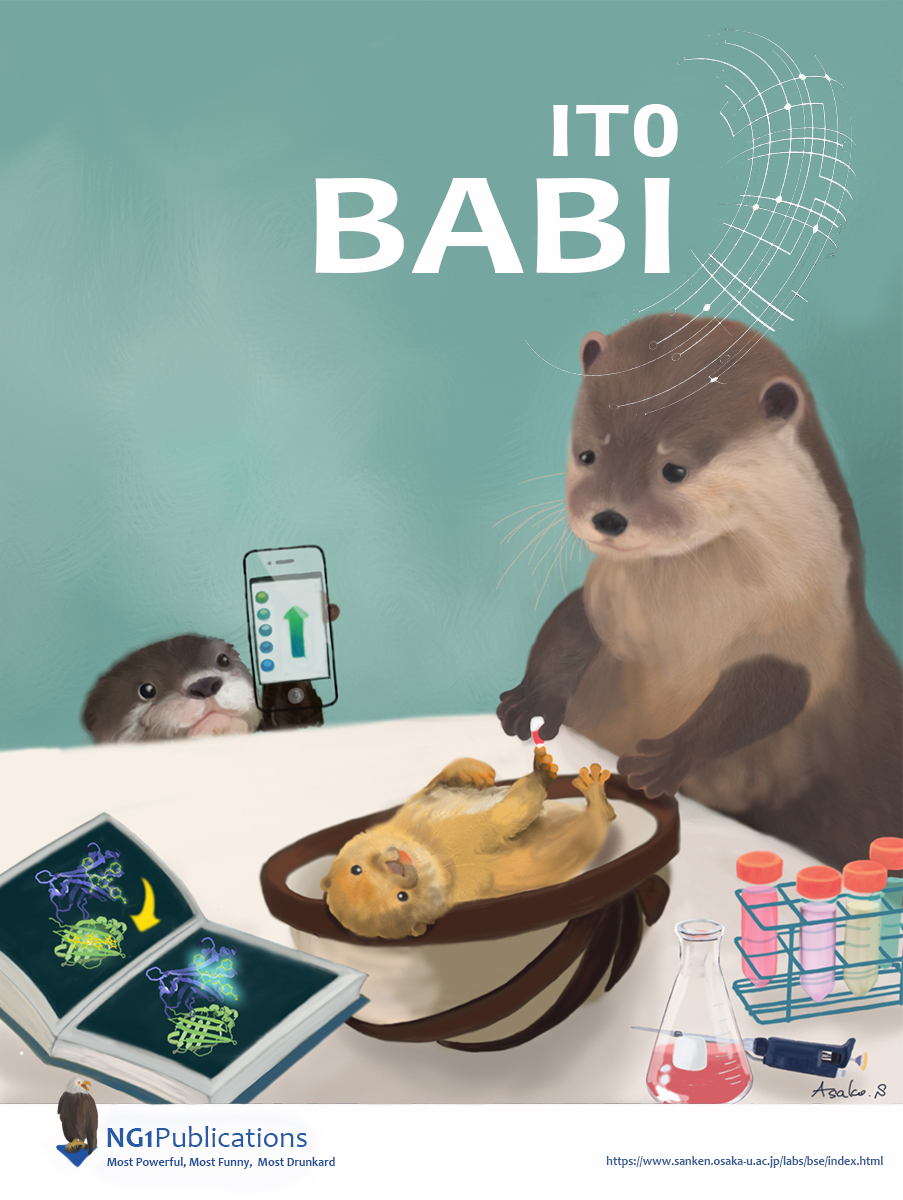
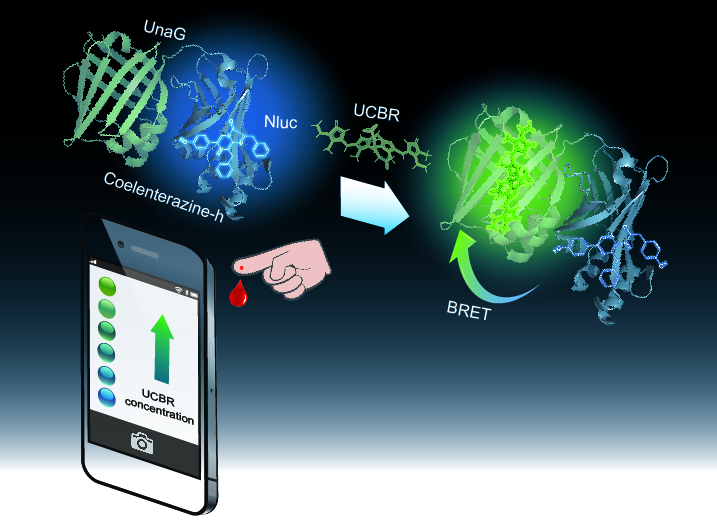
[ LOTUS-V: Genetically encoded bioluminescent voltage indicator for multi-purpose use in wide range of bioimaging ]
( Shigenori Inagaki, Scientific Reports, 9, 7460, 2019 )
Nerve cells form a circuit in our brain, and realize higher-order brain functions such as cognition, action, and memory through the propagation of electrical signals. In order to understand these brain functions, electrodes have been utilized to measure brain activity. However, this technique requires cables to be connected to each mouse, especially when measuring brain activity in animals which move freely. Therefore, if simultaneous measurement was performed from multiple mice performing social behavior, the cable entanglement became a problem which making research still been challenging. Recently, we developed a new brain activity measurement method that using the bioluminescent membrane potential sensor LOTUS-V. By using LOTUS-V to change its emission color according to neural activity, it is possible to measure brain activity that changes in milliseconds. By using bioluminescence, color changes of LOTUS-V can be detected even from a distance, just like shooting firefly light at night. This wireless live brain activity measurement technology enables the simultaneous measurement from multiple free-moving mice which is the first time in the world. Using this measurement method, we observed the brain activity when a mouse actually touches another mouse. Also it’s the first time that the neural activity in the primary visual cortex increased predominantly according to the contact was found.This indicates that the measurement method developed this time is useful as a method to find the novel brain function. Especially, it is expected that the brain functions governing social behavior such as communication between multiple animals, which have been difficult to study, and the contribution to research and treatment of mental disorders such as autism spectrum and interpersonal phobia.
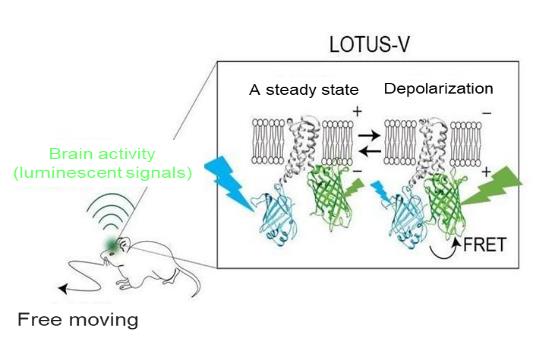
The schematic of LOTUS-V.The measurement of the luminescent color change from the brain domain deploying LOTUS-V. LOTUS-V is a fusion protein consisting of a luminescent protein ( light blue ), a fluorescent protein ( yellowish green ), and a membrane potential sensitive domain ( gray ). When the depolarization is occurred, the membrane potential sensitive domain changes its structure and the length between the florescent protein and luminescent protein gets closer. Then, the FRET efficiency increases and strengthen the yellowish green luminescence ( decreased the light blue luminescence ).
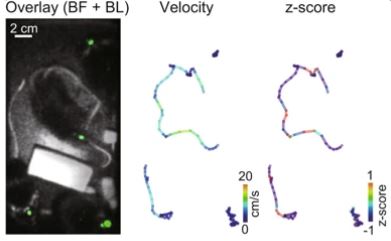
Imaging of interactively moving mice. ( left ) Overlaid image of bright-field and LOTUS-V bioluminescence from multiple mice ( green ). ( middle and right ) Pseudo-colored locomotion trajectory, indicating velocity ( middle ) and z-normalized ΔR/R0 from each mouse ( right ) .
[ Development of five color bright chemiluminescent proteins for real-time multicolor bioimaging ]
( Kazushi Suzuki, Nature Communications, 7, 13718, 2016 )
Luminescence imaging has gained attention as a promising bio-imaging modality in situations where fluorescence imaging cannot be applied. However, wider application to multicolour and dynamic imaging is limited by the lack of bright luminescent proteins with emissions across the visible spectrum. We developed five new spectral variants of the bright luminescent protein, enhanced Nano-lantern (eNL), made by concatenation of the brightest luciferase, NanoLuc, with various colour hues of fluorescent proteins. eNLs allow five-colour live-cell imaging, as well as detection of single protein complexes and even single molecules. We also develop an eNL-based Ca2+ indicator with a 500% signal change, which can image spontaneous Ca2+dynamics in cardiomyocyte and neural cell models. These eNL probes facilitate not only multicolour imaging in living cells but also sensitive imaging of a wide repertoire of proteins, even at very low expression levels.
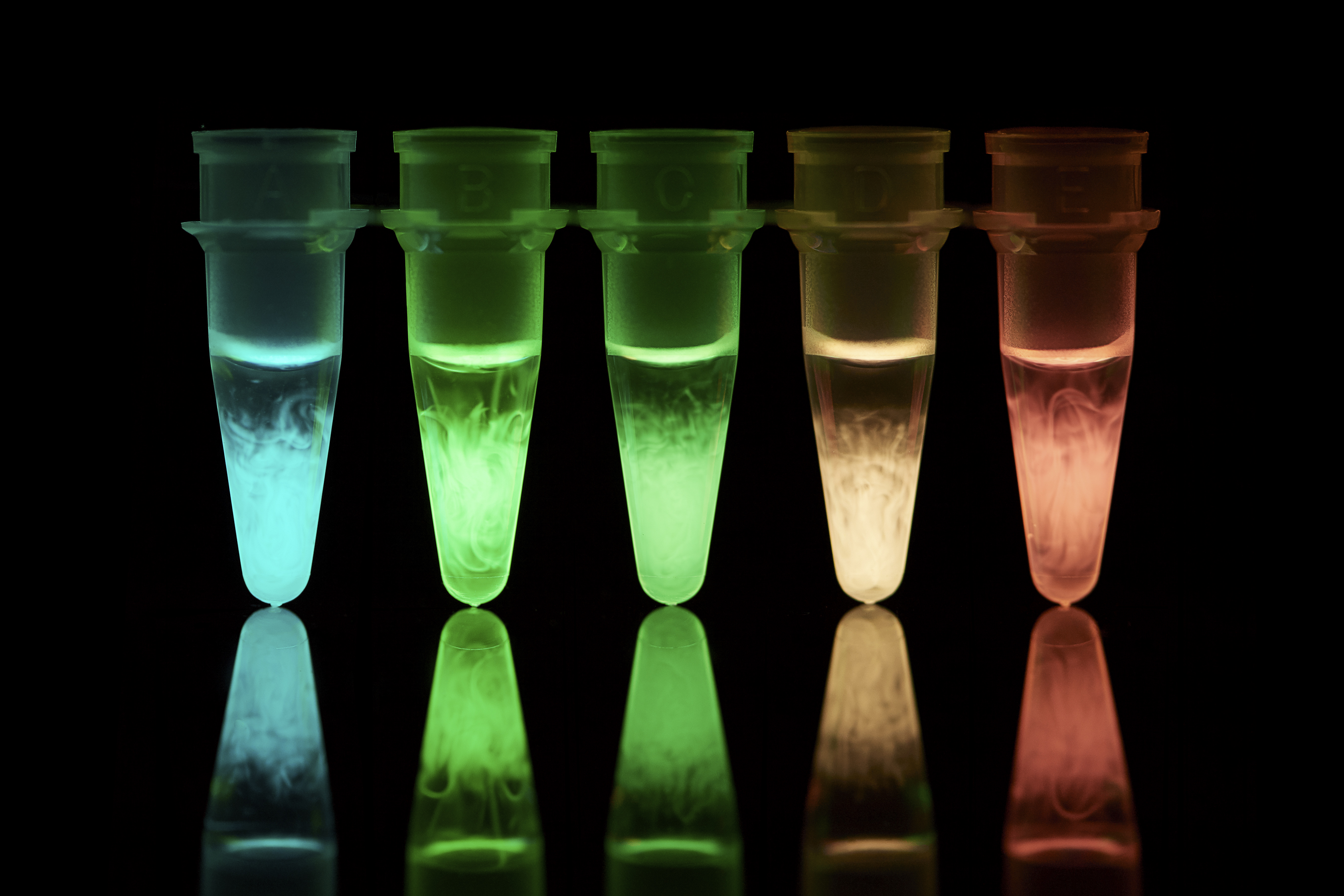
[ Development of cyan and orange super bright luminescent protein ]
( Akira Takai, Proc. Natl. Acad. Sci. USA, 112, 4352-4356, 2015 )
Fluorescence live imaging has become an essential methodology in modern cell biology. However, fluorescence requires excitation light, which can sometimes cause potential problems, such as autofluorescence, phototoxicity, and photobleaching. Furthermore, combined with recent optogenetic tools, the light illumination can trigger their unintended activation. Because luminescence imaging does not require excitation light, it is a good candidate as an alternative imaging modality to circumvent these problems. The application of luminescence imaging, however, has been limited by the two drawbacks of existing luminescent protein probes, such as luciferases: namely, low brightness and poor color variants. Here, we report the development of bright cyan and orange luminescent proteins by extending our previous development of the bright yellowish-green luminescent protein Nano-lantern. The color change and the enhancement of brightness were both achieved by bioluminescence resonance energy transfer ( BRET ) from enhanced Renilla luciferase to a fluorescent protein. The brightness of these cyan and orange Nano-lanterns was ∼20 times brighter than wild-type Renilla luciferase, which allowed us to perform multicolor live imaging of intracellular submicron structures. The rapid dynamics of endosomes and peroxisomes were visualized at around 1-s temporal resolution, and the slow dynamics of focal adhesions were continuously imaged for longer than a few hours without photobleaching or photodamage. In addition, we extended the application of these multicolor Nano-lanterns to simultaneous monitoring of multiple gene expression or Ca2+dynamics in different cellular compartments in a single cell.
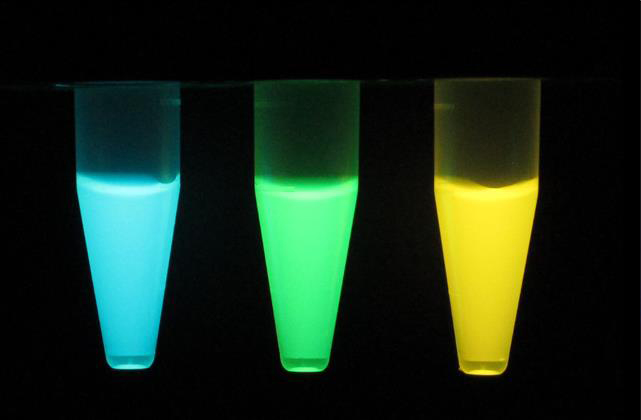
Nano-lantern color variant luminescence
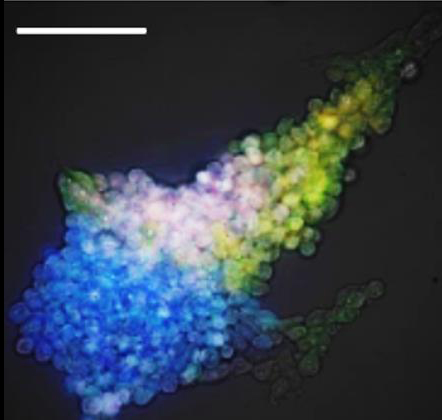
Gene expression analysis by luminescence imaging Scale bar 100 µm
[ Development of ultra bright luminous protein ]
( Kenta Saito, Nature communications, 3, 1262, 2012 )
The use of fluorescent proteins has revolutionized our understanding of biological processes. However, the requirement for external illumination precludes their universal application to the study of biological processes in all tissues. Although light can be created by chemiluminescence, light emission from existing chemiluminescent probes is too weak to use this imaging modality in situations when fluorescence cannot be used. Here we report the development of the brightest luminescent protein to date, Nano-lantern, which is a chimera of enhanced Renilla luciferase and Venus, a fluorescent protein with high bioluminescence resonance energy transfer efficiency. Nano-lantern allows real-time imaging of intracellular structures in living cells with spatial resolution equivalent to fluorescence and sensitive tumour detection in freely moving unshaved mice. We also create functional indicators based on Nano-lantern that can image Ca2+, cyclic adenosine monophosphate and adenosine 5′-triphosphate dynamics in environments where the use of fluorescent indicators is not feasible. These luminescent proteins allow visualization of biological phenomena at previously unseen single-cell, organ and whole-body level in animals and plants.
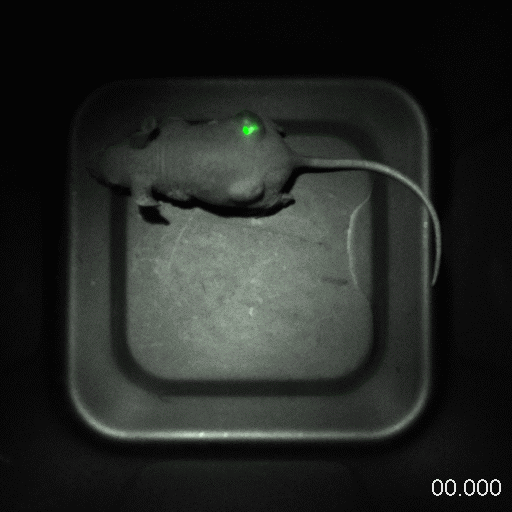
[ Auto-Luminescent Genetically-Encoded Ratiometric Indicator for Real-Time Ca2+ Imaging at the Single Cell Level ]
( Kenta Saito, PLoS ONE, 5: e9935,2010)
It is necessary to apply strong excitation light to the sample in "fluorescence observation" by using FRET or fluorescent protein. Therefore, it is difficult to apply to the inside of organisms where excitation light hardly reaches, or to plants which has high autofluorescence and phototoxic effects. On the other hand, bioluminescent proteins that cause luminescence without requiring excitation light, such as the firefly light, have also been identified and used for research. We are trying to approach 'luminescence observation' which does not require excitation light to imaging to individual organisms. We are also developing biosensor using bioluminescene Resonance Energy Transfer (BRET) from bioluminescent protein to fluorescent protein. As a result, new luminescent Ca2+ indicator BRAC was developed and calcium oscillation of Hela cells expressing was observed.
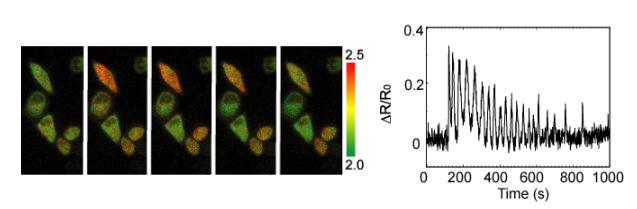
GLICO
Under construction
CeNL( Ca2+ )/OeNL( Ca2+ )
Under construction