[ A photoswitchable fluorescent protein for hours-time-lapse and sub-second-resolved super-resolution imaging ]
( Tetsuichi Wazawa, Ryohei Noma, Shusaku Uto, Kazunori Sugiura, Takashi Washio, Takeharu Nagai, Microscopy, 70, 340–352, 2021 )
Kohinoor2.0, a positively reversibly photoswitchable fluorescent protein, was developed with introducing mutagenesis into Kohinoor ( Dhemendra K Tiwari et al., Nature Methods, 12, 2015 ) Kohinoor2.0 shows 2.6-fold higher brightness, 2.5-fold faster maturation speed, and 1.5-fold faster off-switching speed than Kohinoor. An analysis of pKa of Kohinoor2.0 revealed that the chromophore was under multiple equilibrium and its balance was depended on the brightness. This is the first report that the equilibrium of chromophore of fluorescent protein contributes its brightness, suggesting us a new method to improve the fluorescent protein. With Kohinoor2.0 and SPoD-OnSPAN ( Tetsuichi Wazawa, Microscopy, 67, 2018 ) we successfully observed the dynamics of Actin-filament for 4 h and the dynamics of mitochondria with 0.5 s time resolution in living COS7 cell. In this experiment, we could visualize the intermitochondrial contact, which cannot observe the conventional microscopy. Thus, Kohinoor2.0 would lead us to unveil the intracellular dynamics of fine structure of organelle or specific protein, and more, to visualize the dynamics of functional small molecule such as Ca2+ at high spatial and time resolution.
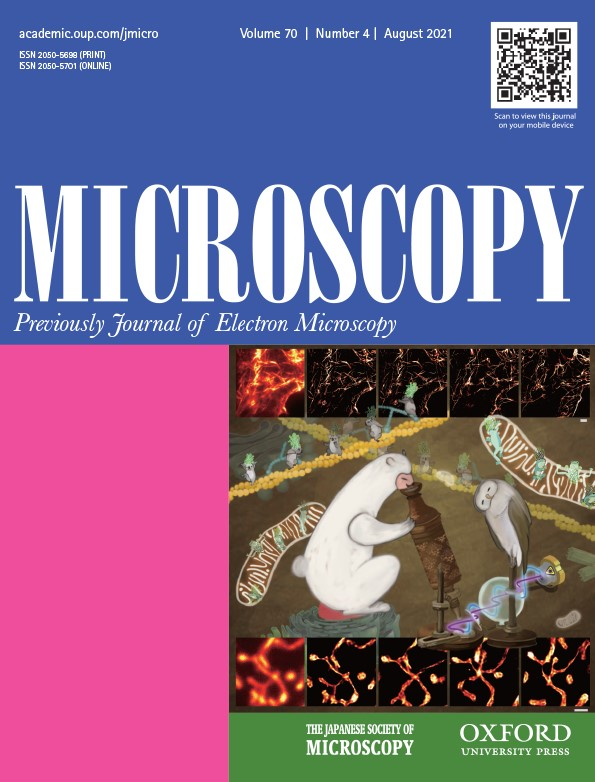
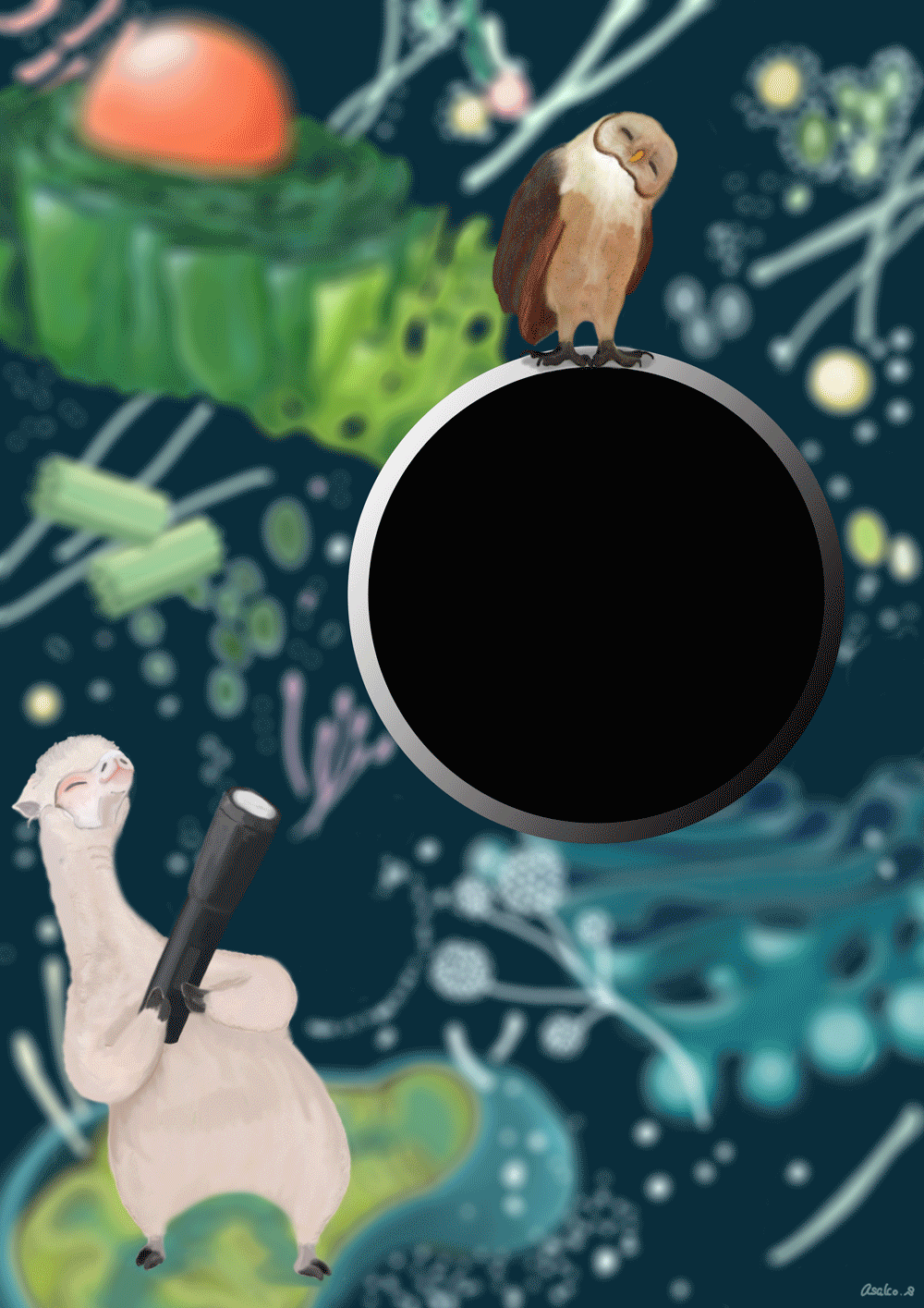
[ Gamillus: Development of acid resistant monomeric green fluorescent protein ]
( Hajime Shinoda, Cell Chem Biol., 25, 3, 2018 )
The fluorescent protein ( FP ) color palette has greatly contributed to the visualization of molecular and cellular processes. However, most FPs lose fluorescence at a pH lower than their neutral pKa ( ~6 ), and this has hampered their application in acidic organelles ( pH~4.5-6.0 ). Currently, several cyan- and red-colored acid-tolerant FPs are available; however, there are few reports of acid-tolerant green FPs ( GFPs ) that are practically applicable to bioimaging. Here, we developed the acid-tolerant monomeric GFP "Gamillus" from the jellyfish Olindias formosa, with excellent brightness, maturation speed, and photostability. Results from X-ray crystallography and point mutagenesis suggest that across a broad pH range the acid tolerance is attributed to stabilization of deprotonation in the chromophore phenyl ring by forming a unique transconfiguration. We demonstrate that Gamillus can serve as a molecular tag suitable for imaging in acidic organelles through autophagy-mediated molecular tracking to lysosomes. Gamillus is a fundamental probe for discovering unknown biological phenomena in acidic environments, which will greatly contribute to medicine and drug discovery research.
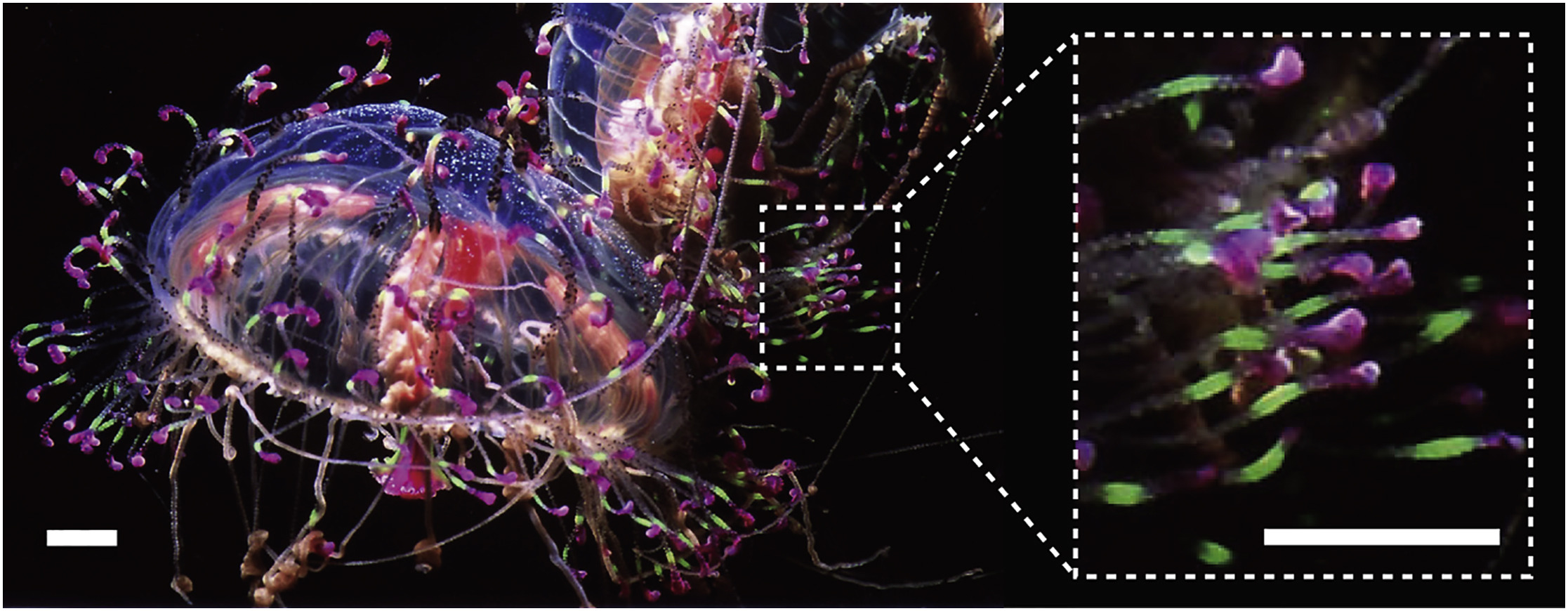
The schematic drawing of Gamillus protein. We cloned the gene of fluorescent protein from Flower Hat Jellyfish's tentacles and improved the brightness and monomeric trait by protein engineering.
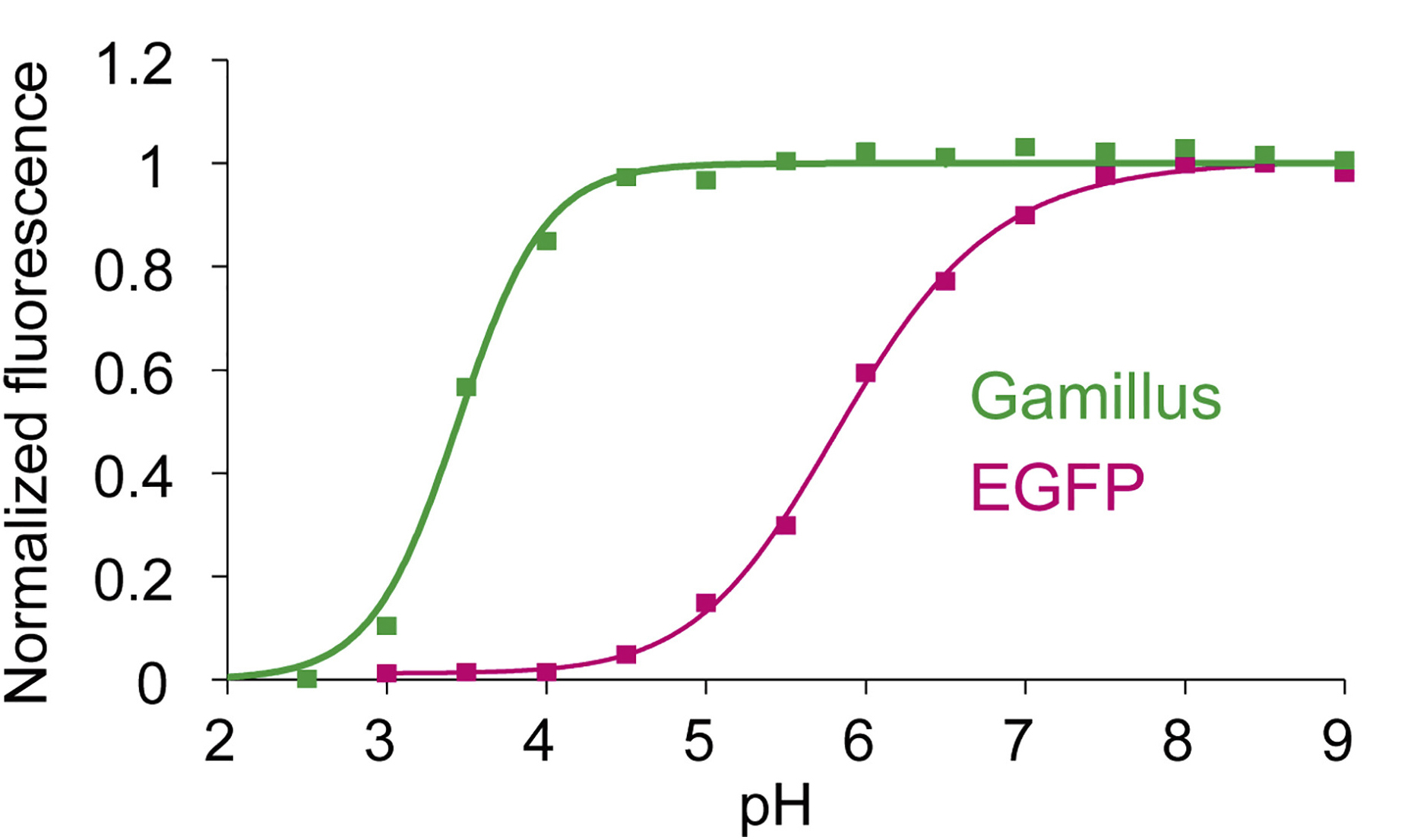
Correlation diagram between pH and fluorescence intensity of fluorescent protein.
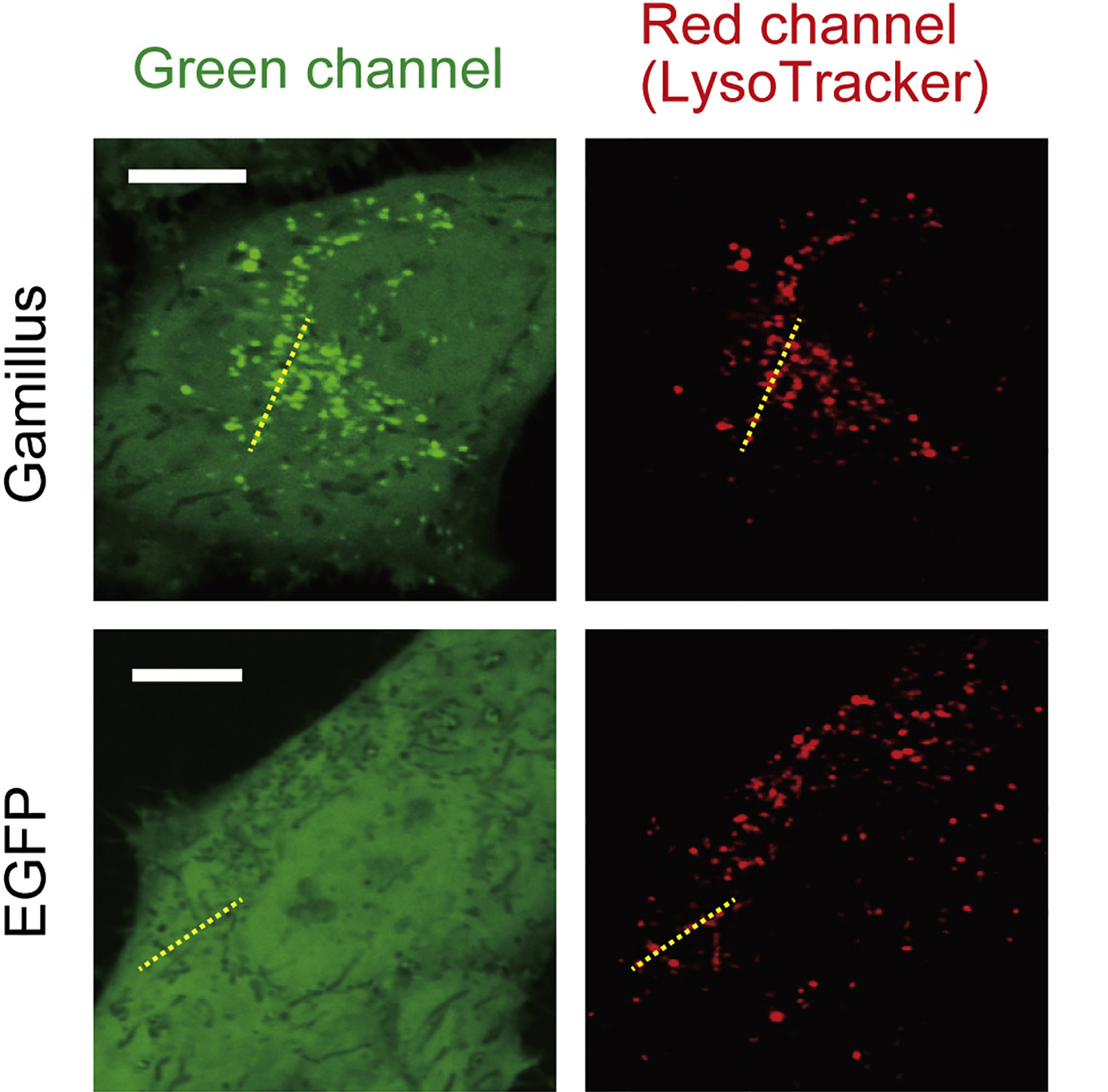
Confocal images of HeLa cells expressing non-fused Gamillus, EGFP, after incubation with LysoTracker red. Images were taken 3 days after transfection. Scale bars, 10µm.
[ Kohinoor: A fast-positive switching, reversibly photoswitchable fluorescent protein ]
( Dhermendra K Tiwari, Nature Methods, 12, 2015 ) [ PDF ]
Light based nanoscopy become key tool to visualize biological structures at sub-nanometer scale, which is not possible by conventional microscopy. Chemistry Noble prize in 2014 for this technique has undoubtly proven high significance in life science research. Most of the nanoscopic methods depend on special photoswitchable fluorescent proteins ( PSFP ), those can be switch-on and off with light. However, the applications of currently available PSFPs are limited in live cell imaging due to photodamage induced by intense light exposure. Our research group developed a reversibly photoswitchable FP ( RSFP ); Kohinoor, with fast positive-photoswitching, high photostability and superior brightness. Kohinoor could be switch-on, switch-off and excited with 488 nm, 405 nm and 488 nm laser, respectively. Kohinoor has fastest switching speed and the highest photostability, which produce ~25 times more switching on-off cycles than the previous positive switching RSFP, Padron. The quantum yield ( QY ) of Kohinoor is 0.71, which is highest among all RSFPs. Kohinoor perfectly expressed to keep many switched on/off repeats with several fusion proteins in various types of cells: HeLa, HEK293, COS7, PC12 and E. coli cells. Thus, Kohinoor is well-suited as a fusion tag for various biological imaging purposes. In reversible saturable optical fluorescence transition ( RESOLFT ) nanoscopy, Kohinoor obtain super-resolved images of vimentin ( ~85 nm ) using a simpler optical setup and much lower laser power than has previously been possible. Therefor Kohinoor has potential in long time live cell nanoscopic imaging to explore cellular dynamics.
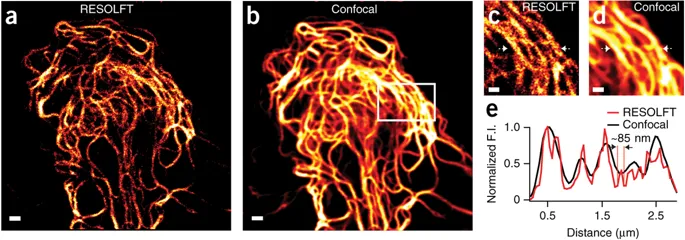
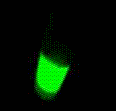
[ SUPERNOVA: Development of fluorescent protein-like “nano-bomb” ]
( Kiwamu Takemoto, Scientific Reports, 3, 2629, 2013 )
Chromophore-assisted light inactivation ( CALI ) is a powerful technique for acute perturbation of biomolecules in a spatio-temporally defined manner in living specimen with reactive oxygen species ( ROS ). Whereas a chemical photosensitizer including fluorescein must be added to specimens exogenously and cannot be restricted to particular cells or sub-cellular compartments, a genetically-encoded photosensitizer, KillerRed, can be controlled in its expression by tissue specific promoters or subcellular localization tags. Despite of this superiority, KillerRed hasn't yet become a versatile tool because its dimerization tendency prevents fusion with proteins of interest. We developed a monomeric variant of KillerRed ( SuperNova ) by direct evolution using random mutagenesis. In contrast to KillerRed, SuperNova in fusion with target proteins shows proper localization. Furthermore, unlike KillerRed, SuperNova expression alone doesn't perturb mitotic cell division. Supernova retains the ability to generate ROS, and hence promote CALI-based functional analysis of target proteins overcoming the major drawbacks of KillerRed.
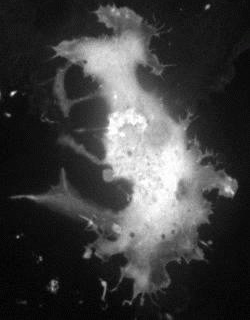
[ PA-TNXL: Development of photoactivatived Ca2+ sensor to enable functional imaging in arbitraty cells in living tissue ]
( Tomoki Matsuda, Scientific Reports , 3, 1398, 2013 )
Genetically encoded fluorescent indicators for bioimaging are powerful tools for visualizing biological phenomena in specified cell types or cellular compartments. However, available gene promoters or localization sequences are not applicable for visualizing all expression events. Furthermore, a visualization technique focusing on single cells or cellular compartments is required for characterizing specific cellular properties including individuality of cells in the cell population. To address these limitations, we developed a genetically encoded caged Ca2+ indicator for which expression timing and location could be controlled. This indicator, PA-TNXL, comprises a Ca2+binding protein and troponin between a photoactivatable FRET donor ( PA-GFP ) and a FRET quencher ( dim variant of YFP ). Ultraviolet irradiation activates the FRET Ca2+ indicator. Using this indicator, we successfully imaged Ca2+ dynamics in a given set of HeLa cells and cultured hippocampal neurons. This technology can be applied for developing other photoactivatable indicators, thereby opening a new area of biological research.
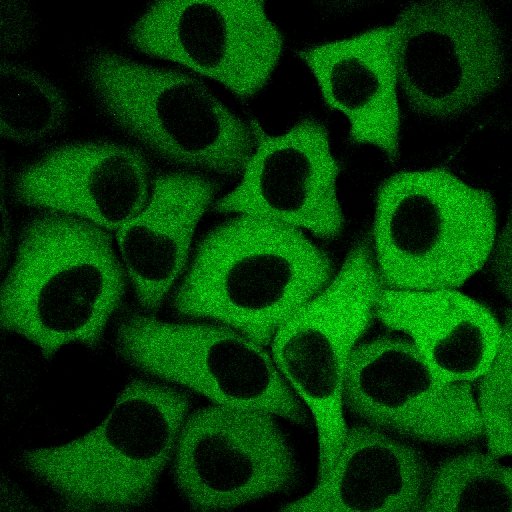
[ Development of blue, green, red calcium ion indicator. An expanded palette of genetically encoded Ca2+ indicators ]
( Yongxin Zhao, Science, 333: 1888-1891, 2011 )
The first fluorescent protein to be known is Green Fluorescent Protein or "GFP" found by Nobel laureate Prof. Akira Shimomura. This GFP is mostly used for fluorescent labeling of cells and biomolecules, and are now widely used for medical and biological research too. In recent years, genetically modified GFP indicators have been developed. These indicators can precisely measure the activation of oxygen in the cell and the ions concentration change. However, the conventional Ca2+ indicator has a disadvantage, it is unable to capture a slight change in Ca2+ concentration because the measurement wavelength range is limited from blue-green to green region and the change in fluorescence intensity caused by Ca2+ bond is too small. In this study, we introduced random mutation into the gene of "GCaMP 3", a green fluorescent Ca2+ indicator using the circularly permutated mutant of GFP. We introduced mutations using "error-prone PCR method" and the gene shuffling method, resulted in development of a green indicator "G-GECO" ( gene fluorescence genetically encoded Ca2+ indicators for optical imaging ) with a signal change rate of 2600%. Furthermore, by substituting "histidine" for "tyrosine" ( which is one of the amino acids ) can change the fluorophore of G-GECO, so it is become possible to replace the blue indicator "B-GECO" with the red fluorescent protein "mApple". Using this technique, we then successfully in developed the red indicator "R-GECO". Subsequently, "Error-prone PCR" was performed using the gene mixture of B-GECO, G-GECO and flash-pericam as a template, and as a result, it was found that "GEM-GECCO" in which fluorescence color changes from green to blue due to binding of Ca2+ is also developed. GEM-GECO shows 11,000% change in fluorescence signal ( blue fluorescence intensity / green fluorescence intensity ) due to Ca2+ binding, this indicator can help to track changes that no previous indicator can do before. In addition, we also developed the indicator with two wavelength excitation measurement "GEX - GECO". The performance of these GECO series was maintained intracellularly and able to observe intracellular Ca2+ fluctuations with extremely high contrast on signal change. In addition, it can also be used to observe Ca2+ in the same cell from multiple compartments, simultaneous measurement of Ca2+ and ATP was also made possible. This research was conducted in collaboration with Robert Campbell of Associate Professor of Chemistry, University of Alberta, Canada, and Professor Ken Ishihara of Bioscience Department of Kyushu University Institute of Science.
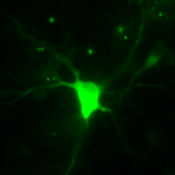
[ Development of the calcium ion sensor “Chameleon-Nano” with the world's highest detection sensitivity
( Kazuki Horikawa, Nature Methods, 7:729-32, 2010 )
Cameleon ( Chameleon ) is a fluorescent protein developed based on genetic engineered GFP using. This has been used as a sensor to detect Ca2+ responsible for intracellular signal transduction real time. So far, some Ca2+ sensors, including chameleons, have been developed by modification of fluorescent proteins and chemical synthesis, but conventional Ca2+ sensors have low sensitivity. This means if there is small amount of Ca2+ usually associated with spontaneous responds can be difficult to measure. We successfully developed of a highly sensitive sensor that can solve this problem. The research group has dramatically improved the avidity to Ca2+ by modifying the Ca2+ binding domain of chameleon. This way lead to the development of the ultra-sensitive Ca2+ sensor “chameleon-Nano”. Cameleon-Nano's binding strength to Ca2+ is the strongest among the Ca2+ sensors developed so far, and it has the world's highest sensitivity ( dissociation constant Kd = 15 nM ). By finely adjusting the Ca2+ binding region, we also developed 5 types of sensors that changed the binding strength to Ca2+, and make the series of sensors. The use of these ultra-sensitive Ca2+ sensors not only makes it possible to detect neural activity in the cerebral cortex with high sensitivity, but also enables neural networks in living animals and muscle tissues controlled thereby. It has become possible to capture phenomena that could not be detected by conventional Ca2+ sensors, such as success in simultaneously measuring both activity patterns.
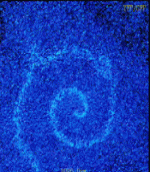
[ Development of ultramarine fluorescent protein ( sirius ),this protein is the shortest wave length fluorescence record of fluorescent protein was updated after an interval of 15 years ]
( Wataru Tomosugi, Nature Methods, 6, 351-353, 2009 )
Green fluorescent protein ( GFP, Green Fluorescent Protein ) for the Nobel Prize in Chemistry in 2008 is discovered from Aequorea victoria by Dr. Osamu Shimomura in the 1960's, develops the gene of GFP in the 1990's, and a lot of fluorescent color variants have been developed by the inheritable genetic modification after the cloning. Many fluorescent proteins that originate longer-wavelength-fluorescence than green fluorescence, such as yellow, orange, and red fluorescence, were developed. However, few fluorescent proteins that originate shorter-wavelength-fluorescence, such as purple and blue fluorescence were developed. Therefore, the development was being demanded by a lot of researchers for years. Our group succeeded in developing fluorescent protein, Sirius, that originated ultramarine fluorescence between purple and blue by introducing the mutation to the chromophore of the fluorescent protein and surrounding it, and furthermore, a random amino acid mutation to the entire protein, then the shortest wave length fluorescence record of the fluorescent protein was updated after an interval of 15 years. As a result, the variation of the fluorescent color increased, therefore, we could visualize the two or more parts of cell simultaneously and more clearly. Sirius is insensitive to pH change, that is, Sirius yield fluorescence under any pH condition unlike ancient fluorescent proteins, therefore, the observation of the movement of the protein in acidic subcellular organelle became possible. We also found that FRET ( Förster resonance energy transfer ) from Sirius to CFP come about effectively because fluorescence spectra of Sirius overlaps with absorbance spectra of CFP considerably. By using both Sirius-CFP FRET pair and uvGFP ( excited by UV light and originates green fluorescence )-DsRed ( red fluorescence ) pair simultaneously, we visualized four colors fluorescence with one excitation wavelength ( Dual FRET method ). It became possible to catch two physiology phenomena simultaneously by using this Dual FRET method, and the road where the relations between two or more physiology phenomena were analyzed in living cells opened. Figure shows the activation of caspase 3 that happens by the program cell death of HeLa cell ( upper ) and Ca2+ dynamics ( lower ) in single living cell.
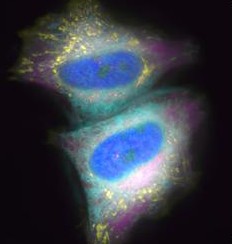
[ Visualization of molecular dynamics in live cells with molecular designed photoconvertible fluorescent protein ]
( Tomoki Matsuda, Nature Methods, 5, 339-345, 2008 )
With the progress of the live imaging technology using fluorescent proteins, today photoconvertible fluorescent proteins, which can change the fluorescent color by irradiation of photostimulation light such as UV light, are used to analyze dynamics of molecules or cells. Previously, observation of fluorescent wavelength change of photoconvertible fluorescent proteins required two different wavelength lights for excitation. Our laboratory designed a chimeric protein by fusing two fluorescence proteins to observe fluorescence of before and after photostimulation (before: cyan; after: green) by one excitation light and developed photoconvertible fluorescent protein “Phamret”. Since only one excitation light is needed, complicated optical system is unnecessary for simultaneous imaging of two fluorescent light caused by photostimulation. Therefore imaging of fast-moving molecules became possible. Another advantage of one excitation light is decreasing phototoxicity for photosensitive creatures. By using photoconvertible property of “Phamret”, we also developed the method to determine the diffusion coefficient “FDAP”. In this method photoconversion is done in the limited region and fluorescent decay caused by outflow of photoconverted molecules is measured. Diffusion coefficient is determined by numerical analysis of the fluorescent decay curve. By using this method easy and precise determination of diffusion coefficient for fast moving molecules is possible compared with conventional methods such as the method analyzing fluorescence recover after photobleaching. New insight into the mechanisms of signal transduction, development of cells or individuals, or metastasis or invasion of tumor cells are expected to be obtained by making use of these methods.
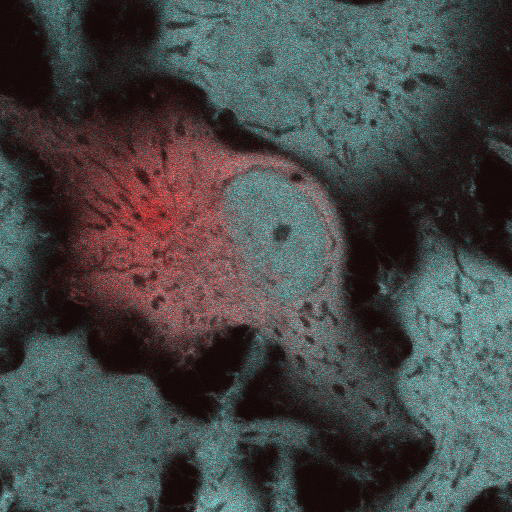